QUASAR R&D at IPAC22 - Detectors and Diagnostics
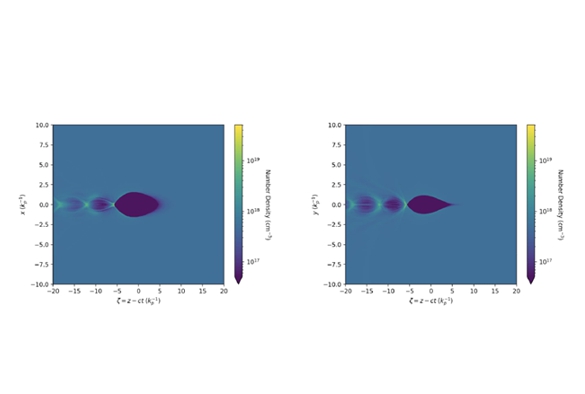
This year the QUASAR Group contributed with a number of original research articles to IPAC 22. Below is a summary of the Group activities related to the design and development of diagnostics techniques
One of the contributions was related to the upgrade of Digital Tomosynthesis (DT) technology. DT is a medical imaging process that offers 3D image quality comparable to computed tomography but at a fraction of the dose. The Group presented a Monte Carlo simulation framework and a computer-aided design software that can reveal the optimum X-ray source and integrated system architecture for a robust, compact, and high image quality multi-X-ray source digital tomosynthesis system [1].
Irradiation geometry for chest Digital Tomosynthesis using: (a,b) a single source array and (c) multiple source arrays. (Image credit Thomas G Primidis et al 2022 Biomed. Phys. Eng. Express 8 015006, CC BY 4.0)
As accelerators become more complex and deliver higher quality bunches, charged particle detection became an important research area. In particular, the ability to image the beam halo, a low-intensity region of particles surrounding the central beam core, is important as the growth of the halo limits the accelerator performance.
Gas-Beam interaction captured on the phosphor screen (Image credit: Oliver Stringer)
The Group demonstrated that a thin supersonic gas can be used to image the beam halo relying on the ionization and fluorescent interactions which occur when hitting the beam. This discovery is currently developed as a gas-jet beam profile monitor, being one of the first non-invasive diagnostics technologies used for halo detection [2].
Beam-based plasma accelerators operate in the blowout regime. This is a situation in which the incoming electron bunch repels plasma electrons creating a bubble of unbalanced ions, virtually void of background electrons. In electron beam facilities like FACET-II and Argonne Wakefield Accelerator facility, beams with highly asymmetric emittance are of interest because they are the preferred type of beam for linear colliders. The Group modelled various scenarios including a weak blowout and a strong blowout.
Electron density plots show that different blowout configurations are formed due the asymmetry of the incoming beams. (Image credit: Monika Yadav)
The radiation patterns generated by these beam-plasma interactions were studied and assessed for their potential use towards a new diagnostics technique [3].
An important part of the gas jet diagnostics developed the the Group is the characterisation of gas jets before interacting with the particle beam. A modified Nomarski interferometer was proposed and designed using a Wollaston prism to create the interferogram. The system uses interchangeable nozzle diameters ranging from 30 μm to 1 mm to allow for testing across a range of gas jet sizes.
Interferometer components. (Image credit: C. Swain et. al., 2022, 13th Int. Particle Acc. Conf., doi:10.18429/JACoW-IPAC2022-MOPOPT054.)
The scheme uses a 532 nm laser which passes through the gas jets, sampling the fine structure of the droplets, and then through the Wollaston prism before ending on a complementary metal–oxide–semiconductor camera [4].
The CERN Linear Accelerator produces electron beams from a photocathode coated with cesium telluride (Cs₂Te). The Group was involved in experimental work aimed to study the linearity and response time of the diamond detectors installed on this linac. Apart from having a high radiation tolerance, these detectors are capable of unprecedented nanosecond resolution and allow the study the beam loss mechanisms bunch by bunch.
Experimental setup at CERN Linear Accelerator. Image credit: Sara Morales Vigo
The study addressed the signal linearity, its response function and the signal build-up from multi-bunched beams, revealing saturation effects and temporal distortions. The signal overlap from multi-bunched beams was also analyzed, opening the door to the development of new correction mechanisms for the resulting bunch-by-bunch beam loss signals [5].
- Design and Optimisation of a Stationary Chest Tomosynthesis System with Multiple Flat Panel Field Emitter Arrays: Monte Carlo Simulations and Computer Aided Designs https://doi.org/10.18429/JACoW-IPAC2022-THPOMS033
- A Gas Jet Beam Profile Monitor for Beam Halo Measurement https://doi.org/10.18429/JACoW-IPAC2022-MOPOPT055
- Radiation Diagnostics for AWA and FACET-II Flat Beams in Plasma https://doi.org/10.18429/JACoW-IPAC2022-WEPOST042
- A Modified Nomarski Interferometer to Study Supersonic Gas Jet Density Profiles https://doi.org/10.18429/JACoW-IPAC2022-MOPOPT054
- Linearity and Response Time of the LHC Diamond Beam Loss Monitors in the CLEAR Beam Test Facility at CERN https://doi.org/10.18429/JACoW-IPAC2022-MOPOPT046