Radiobiological effectiveness of protons
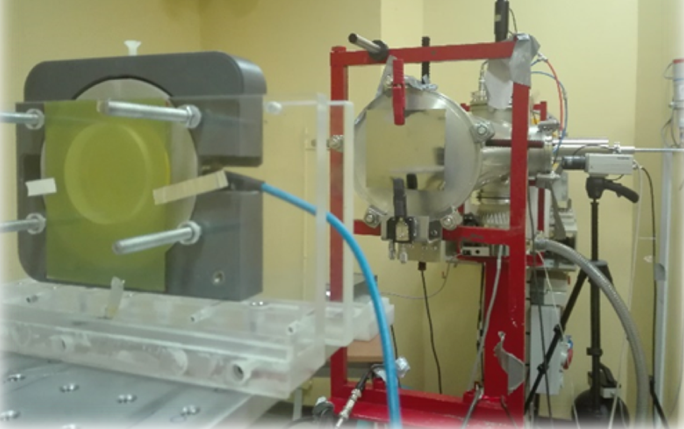
OMA Fellow Anna Baratto Roldán, will present in August 2018 her first report on the status of her project “Radiobiological effectiveness of protons”. This project aims to set up a new system for the irradiation of biological samples at the IBA cyclotron installed at the National Centre of Accelerators in Seville (Spain). This cyclotron is mainly used for the production of radioisotopes for Positron Emission Tomography (PET), but it is also equipped with an external beamline for interdisciplinary research, producing an 18 MeV proton beam or a 9 MeV deuteron beam.
The rationale behind Anna’s project is given by the necessity of performing studies of proton Relative Biological Effectiveness (RBE) at low energies, which would help reaching a consensus on the variation of proton RBE near the Bragg peak. In current clinical practice, the RBE of protons is considered to have a constant value of 1.1, which means that protons are considered to be 10% more effective than photons [1] in the determination of the same biological effect. The use of a constant RBE value is an approximation, generally supported by the fact that the available biological data are insufficient to justify clinically the use of other proposed approaches [2-3]. There is, however, a large amount of data demonstrating that proton RBE is a complex variable, which depends on many factors, including tissue type, biological endpoint, dose and radiation [4], and which varies towards the distal Bragg peak region. Therefore, not taking into account the variations in RBE along the proton beam may have important clinical consequences [2-3][5].
To perform studies of proton RBE at low energies, dedicated beamlines are required, for which special beam optimization and dosimetric techniques are needed. At the National Centre of Accelerators (CNA) beamlines of this kind are available at the 3 MV tandem and the 18 MeV cyclotron facilities, which can be adapted to allow reliable measurements and cell irradiations at low proton energies. The spatial configuration of the two beamlines allows the irradiation of cells, growing in monolayer cultures placed in Petri dishes and mounted at the exit of the beamline, orthogonally with respect to the beam axis. With this configuration, two are the major constraints when irradiating biological samples: low beam intensity, of the order of some pA, to control properly the fluence within suitable irradiation time scales; and the irradiation field, which has to be broad enough, of the order of few cm to cover the whole sample, and homogeneous in both energy and spatial distribution.
In the case of the 18 MeV cyclotron external beamline, a simple beam line diagnostic and irradiation scheme, based on a completely defocused beam, external scattering foils and an ionization chamber coupled with EBT3 radiochromic films, has been used for the determination and measurement of the optimal beam parameters for radiobiology experiments. Measurements of the intensity profile have been performed using EBT3 radiochromic films and a transmission ionization chamber for proton fluence evaluation. Experiments have been done in different irradiation conditions, degrading the beam with tungsten scattering foils of different thicknesses, from 50 to 200 µm, and varying the distance between the exit window and the sample. A homogeneous irradiation field, with maximum deviations around 8% in the whole sample area (3.5 cm of diameter), has been achieved for a beam energy of around 11 MeV and below, and with a feasible beam intensity. In parallel, Monte Carlo simulations of the beamline have been performed with the SRIM and Geant4 codes, to be compared and matched with experimental data. These simulations reproduce quite well experimental data in what concerns beam profiles measured with EBT3 radiochromic films, with maximum deviations of about 13% and 9%, respectively. Future improvements of the beamline are still under study; different solutions such as changes in the collimation system or the installation of a system for the dispersion of the beam inside the beamline vacuum pipe are currently being considered.
References
[1] International Commission on Radiation Units and Measurements, Prescribing, Recording and Reporting Proton-Beam Therapy, (ICRU Report 78), J ICRU (2007); 7.
[2] G. Giovannini et al., Variable RBE in proton therapy: comparison of different model predictions and their influence on clinical-like scenarios, Radiation Oncology (2016); 11:68.
[3] A. Carabe et al., Range uncertainty in proton therapy due to variable biological effectiveness, Physics in Medicine and Biology(2012); 57:1159-1172.
[4] H. Paganetti, Relative biological effectiveness (RBE) values for proton beam therapy. Variations as a function of biological endpoint, dose, and linear energy transfer, Physics in Medicine and Biology (2014); 59:R419-R472.
[5] M. Wedenberg et al., Disregarding RBE variation in treatment plan comparison may lead to bias in favor of proton plans, Medical Physics (2014); 41:091706.
This work is related to OMA deliverable 2.14