New Article Published on Terahertz Radiation
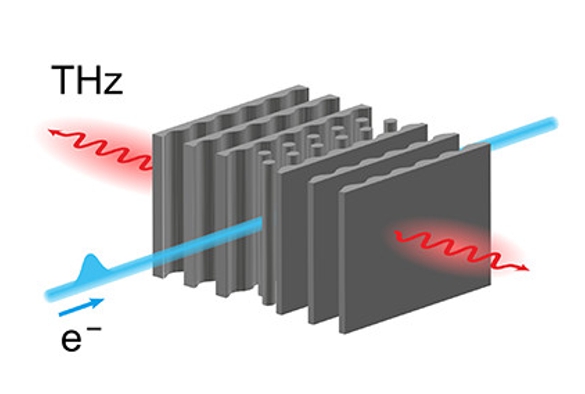
The terahertz (THz) radiation domain spans between the microwave (MW) and the infrared (IR) regions on the electromagnetic spectrum. Previously, unexplored due to the lack of suitable electronic, various disciplines are nowadays exploring and making use of the of THz radiation. Examples are medical imaging, material research and communication. Recently electron acceleration by THz radiation was demonstrated in a paper published in Nature. Among the several methods to generate THz radiation, the Smith Purcell effect provides a compact and cost-efficient alternative. The effect is observed as radiation emitted from charged particles travelling in the proximity of periodic gratings or periodic material inhomogeneity.
The University of Liverpool's QUASAR Group, including LIV.DAT student Gyanendra Yadav, joined a team of researchers from Paul Scherrer Institute and Friedrich-Alexander-Universität to work on a new concept of using THz radiation for electron acceleration. The work published in ACS Photonics outlines a technique called “Inverse-Design” to optimize a structure for generating narrowband Smith-Purcell radiation. More exactly corrugations on a narrowband dielectric structure were numerically tuned to stimulate and maximize the Smith-Purcell radiation for a 3.2 GeV electron beam.
The optimization was based on a 2D finite-difference frequency-domain (FDFD) simulation of a single unit cell of the grating. Periodic boundary conditions were imposed along the propagation direction of charged particles. Dielectric material was chosen for the grating structure instead of conventional metallic gratings used in Smith Purcell radiators.
An electron beam (blue) passes along the surface of inverse-designed dielectric structure emitting Smith-Purcell radiation (red).(Image credit: Benedikt H, et al., ACS Photonics Article ASAP License: CC BY-NC-ND 4.0)
Dielectrics have a field-induced damage threshold 1-2 orders higher than metals which makes them an attractive choice of material for this purpose. The grating structure was fabricated at Paul Scherrer Institute (PSI) by using a laser-based 3-D printer for polymers with a design wavelength of 900 µm. A high-temperature resin was used as a dielectric material as it had high vacuum compatibility after being cured in a heated vacuum chamber. The 45 mm-long and 6 mm-high structure was then placed into the ACHIP experimental vacuum chamber at SwissFEL. The electron bunch length was compressed to 30 fs and the measured transverse beam size in the vertical direction was 40 µm and 30 µm in the horizontal. A Michelson interferometer was assembled outside the vacuum chamber for spectrum measurements.
Emission spectra. The Fourier transform of an autocorrelation measurement with a Michelson interferometer (black) is compared to 3D time-domain (green) and frequency domain (orange) simulations. The gray area indicates the acceptance window of the spectrometer, defined by the angular acceptance of the Michelson interferometer. (Image credit: Benedikt H, et al., ACS Photonics Article ASAP License: CC BY-NC-ND 4.0)
The image above shows the experimentally observed and simulated emission spectra. The experimental spectrum (black) is in good agreement with 3D simulated FDTD spectra (green). Another FDFD simulation (orange) shows the theoretical possible narrowband spectra which could be possible acquired by this radiator. The difference in the two simulated spectra appears due to the different periodicity of the grating structures used. Both measured and time domain simulated spectrum has a peak wavelength around 881 µm. The energy of the radiation was measured to be 0.6 pJ for approximately 11 pC of bunch charge and it can be increased by a factor of 300 by increasing the bunch charge to the available 200 pC at SwissFEL.
The experimental spectrum is limited due to the structure fabrication resolution of 140 µm. Similarly, the FDTD simulation is limited by the computational resources as its time-domain solver calculates the electromagnetic field for every time step for the whole 50-period long grating structure, making it a better reproduction of the experimental spectrum.
This work concludes with the fact that the inverse designed algorithmic method of geometry optimization can produce narrowband and beam synchronous THz radiation and could advance the THz driven pump-probe investigations at free-electron lasers. Moreover, the design can be adjusted to serve as a radiation source for any arbitrary wavelength which is harder to produce by other methods. It also paves the way for future on-chip compact devices with integrated electron sources.
Further information
'Inverse-Designed Narrowband THz Radiator for Ultrarelativistic Electrons'
Benedikt Hermann, Urs Haeusler, Gyanendra Yadav, Adrian Kirchner, Thomas Feurer, Carsten Welsch, Peter Hommelhoff, and Rasmus Ischebeck
ACS Photonics Article ASAP
DOI: 10.1021/acsphotonics.1c01932
License: CC BY-NC-ND 4.0 https://creativecommons.org/licenses/by-nc-nd/4.0/