ATLAS Experiment uses new data collection techniques to study ‘Little Bangs’
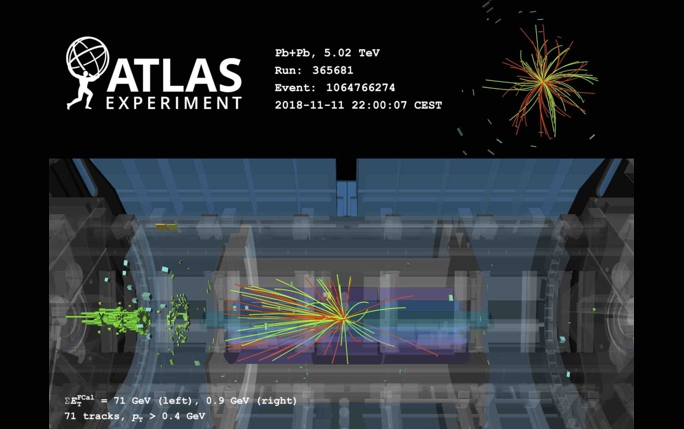
A new result from the ATLAS Collaboration studies the interactions of photons (i.e. particles of light) with lead nuclei at the Large Hadron Collider (LHC). Using new data collection techniques, physicists revealed that these exotic collisions dubbed ‘Little Bangs’ show an unexpected similarity to the experimental signatures of the quark–gluon plasma.
ATLAS is a general-purpose particle physics experiment designed to exploit the full discover potential of the LHC at CERN, using precision measurements to push the frontiers of scientific knowledge. Its collaboration is one of the largest in science with over 5000 members from around the world.
Two of these members are LIV.DAT PhD student Adam Ruby and his supervisor Dr Nikolaos Rompotis. Adam analyses data detected by the ATLAS detector to search for rare Higgs decays involving a dark matter candidate known as Axion. For his project, he explores how machine learning and big data methods can improve the sensitivity for discovering new particle decays from data collected in data collection period known as Run-II.
An LHC configuration with colliding lead nuclei gives physicists an opportunity to study the quark–gluon plasma (QGP), an intensely hot and dense phase of matter that is created when the nuclei collide head-on. These extreme conditions mimic those of the early Universe during the first micro-seconds after the Big Bang.
In a recent publication, ATLAS physicists were surprised to see that some of the most energetic photonuclear collisions – a high-energy photon striking an on-coming photon from another nucleus – showed flow patterns similar to those observed in hot and dense QGP observed in head-on lead–lead collisions. Specifically, the particles exhibited an azimuthal momentum anisotropy in the transverse plane. This signature is traditionally interpreted as evidence of QGP formation. The overall data offer a tantalising suggestion that quark–gluon plasma may be formed even in these exotic, small collision systems.
Most theoretical models of these momentum anisotropies rely on the colliding bodies being made of quarks and gluons. Naively, it is surprising to find such effects in a system where one of the colliding particles is a simple, structureless photon. However, at large enough energies, the photon’s wave function is a superposition of many states, including some that are hadrons (particles composed of quarks and gluons). Thus, these measurements provide a collision system with a very different initial structure than those traditionally used to study the quark–gluon plasma – and serve as a test for experimentalists and theorists alike.
As part of this research, Monte Carlo (MC) simulations of the relevant physics processes were used to understand the performance of the detector, and provide distributions to be compared with the data. MC methods are powerful tools and a number of work packages within the Liverpool Big Data Science CDT focus on this – MC calculations allow comparison of theoretical models with the large and complex experimental data sets typically produced by experiments in astronomy, nuclear physics and particle physics.
To read more about these new results, the full update by the ATLAS experiment can be found here.
Full article:
The ATLAS Collaboration., G. Aad, B. Abbott, et al., Two-particle azimuthal correlations in photonuclear ultraperipheral Pb + Pb collisions at 5.02 TeV with ATLAS, Phys. Rev. C 104, 014903 – Published 12 July 2021
https://doi.org/10.1103/PhysRevC.104.014903
Image: A photonuclear collision, where a high-energy photon strikes a lead nucleus. The charged particles that bend in the magnetic field are shown as lines and energy deposits in the calorimeters (green and blue blocks). In this photonuclear collision, the participating lead nucleus was going to the left, while the photon was going to the right (Image credit: ATLAS Collaboration) - https://cds.cern.ch/record/2775156